What is a turbocharger?
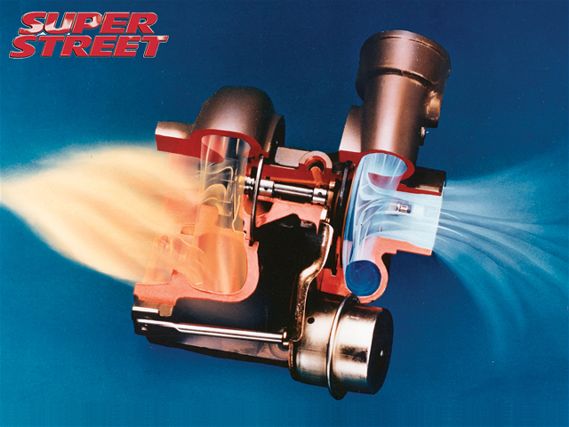
A turbocharger consists of two separate devices linked by a common shaft: A centrifugal supercharger and a gas turbine. The supercharger's compressor fan--with greater pumping capacity than the engine it is boosting--forces air into the intake manifold at higher than atmospheric pressure--providing extra oxygen so the engine can burn much more fuel and thus produce dramatic increases in power and torque. Meanwhile, the turbine harnesses exhaust gas pressure and heat against jet-like fan blades, providing the shaft horsepower and torque needed to drive the compressor beyond the 40,000 or so RPMs typically required to make measurable boost.
The smallest Garrett turbochargers safely rotate up to as much as 250,000+ RPMs, with many larger automotive units toping out at a little over 100,000 RPMs. Larger-sized turbos--like larger jet engines--tend to be a bit more efficient.
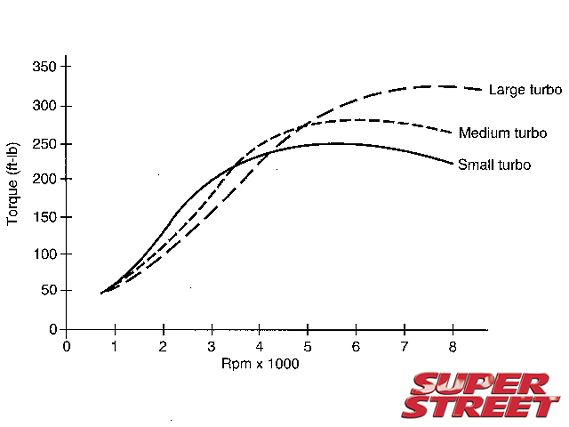
As the compressor "spools up" and begins to create boost, the engine starts to burn more fuel and create more exhaust--which spins the turbine faster, which spins the compressor faster, which creates more boost, which creates more exhaust, and so on--a self-feeding cycle in which--to a degree--the more power you make, the more power you can make. The time it takes to accelerate the turbine and compressor to operating speed and make boost when you put the pedal to the metal, is known as turbo lag. Careful design can effectively eliminate turbo lag as a practical consideration, although there may then be a tradeoff in performance at peak power.
A turbo compressor is precisely analogous to a centrifugal supercharger like the Vortech, which is scaled up in size and fitted with radial (rather than reverse-curved) blades for increased pressure ratio at lower compressor speeds (at the cost of efficiency and a narrower operating envelop) and powered off the crankshaft. The turbo's centrifugal compressor and turbine each consist of a superficially-similar centrifugal fan freewheeling within a scrolled housing. The inlet side ("inducer") of the compressor fan is smaller than the outlet ("exducer") side that flings air into the compressor housing and discharge, and vice versa for the turbine.
The "cold-side" compressor housing is machined from cast aluminum, while the turbine's "hot housing"--which must survive temperatures that can approach 2000 degree F--is made of thick, machined cast iron. The compressor and turbine wheels are attached to a common shaft, with a housing in the center locating the shaft on bushings or ball bearings that are lubricated with high-pressure engine oil and may also be cooled with radiator fluid pumped through the center section close to the bearings. Oil drains back into the oil sump via gravity, while the engine's water pump circulates coolant from the turbo back to the radiator for cooling.
It takes about 30 horsepower to generate 100 horsepower worth of boost. When a centrifugal compressor is driven by a belt or gears from the crankshaft, the 30 HP is wasted in the form of parasitic drag. Not so on a turbocharger: Eighty percent of the energy that drives a turbocharger's turbine comes from exhaust gas heat that would otherwise be wasted out the tailpipe; the remaining 20 percent comes from exhaust pressure which does have a cost in the form of decreased pumping efficiencies resulting from higher backpressure in the exhaust manifold. Therefore, the "cost" of a turbo conversion is only 5-10 horsepower out of 100 gained rather than 30. Bottom line, turbochargers are simply more efficient power-adders than blowers or nitrous, which is why turbo engines dominate all forms of racing where they are allowed to compete without crippling sanctions.
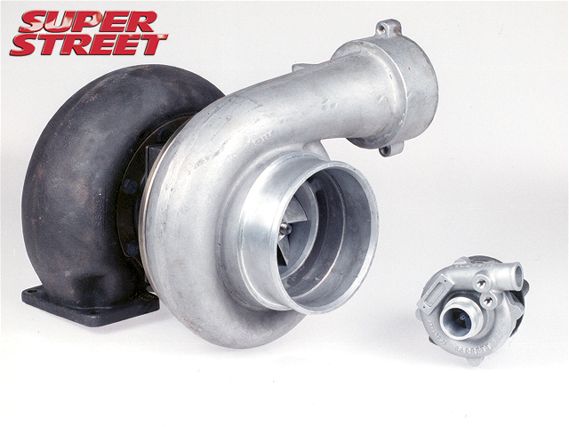
Turbochargers, like jet engines or dogs, come in a bewildering variety of sizes, from miniature units that easily fit in the palm of a human hand (suitable for boosting a tiny motorcycle engine) to gigantic fixtures as big as a spare truck tire used to increase the power output of a 6,000 HP locomotive or even a 25,000 HP container ship. The size and operating characteristics of a compressor and turbine can be mixed and matched on a turbocharger to optimize performance for a specific application.
Turbo Pathologies
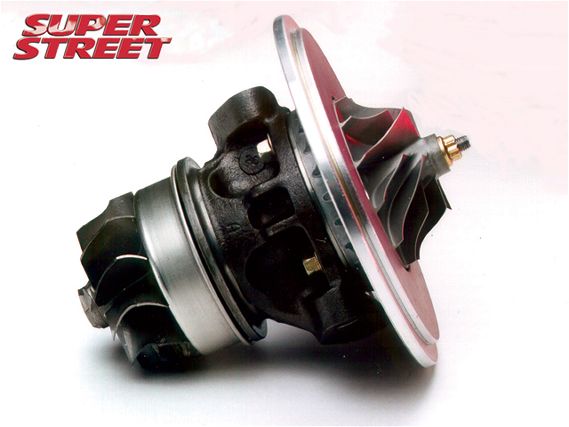
Garrett engineer Jason Kavanagh points out that a lot of turbo application "no-nos" can damage bearings: "Since ball-bearing turbos (well, Garrett's anyway) have many times the load capacity of conventional bearing systems, they can cover a lot of these sins. Coupled with their superior transient boost response, this increased robustness has made Garrett BB turbos very popular in the aftermarket." Bottom line, sizing issue can definitely affect turbo longevity.
1) Oversized compressor wheel for an engine. The most notable symptom will be audible compressor surge when boost is coming up. Surge can damage bearings. Compressor surge in this manner is often accompanied by a lumpy power delivery. Boost response will also suffer relative to a suitably sized compressor.
2) Undersized turbine housing (too small A/R) will give more immediate boost response, to the point of jumpy boost response at throttle tip-in. It will also backpressure the engine a lot, which will make the power go flat early in the rev range, make the engine more detonation-sensitive, and in extremis more likely to overheat.
3) Oversized compressor wheel and undersized A/R will tend to exacerbate the compressor surge by making it come up on boost quicker. Surge can damage bearings. On the plus side the boost will come up quicker...
4) Undersized turbine wheel and large turbine A/R "Hard to say in general terms; depends on other factors (choice of engine, compressor)"
5) Undersized compressor wheel Good boost response but power will go flat earlier in the rev range because comp efficiency drops like a brick as you approach choke (which is what happens when a compressor is undersized for an engine), and because backpressure will be climbing up (the turbine has to do this to keep up with the plummeting compressor efficiency). When wound to redline you also run the risk of overspeeding the turbo and putting excessive loads on the thrust bearing. Also the compressor oil seal will tend to leak.
6) Hybrid turbos (say T3/T04) can work well as long as the wheel diameter mismatch isn't too extreme, and if the wheel aero is good. Some hybrids perform quite well. Some hybrids suck, though.
7) Compressor surge/stall With oversized compressors, surge can occur when boost is coming up, or when the throttle is closed when boost is up. The former case is harder on the thrust bearing, but neither one is really desirable. Surge can happen even if the compressor is sized correctly for the engine--surge can occur if the compressor inlet is too restrictive, and/or if there is a lot of pressure drop in the intercooler plumbing. Also see #1 above
Pumping Up the Boost: Everything Depends on Everything Else and Nothing Is Certain
The pumping and spooling capability of a centrifugal supercharger driven by an exhaust turbine is so complex that you'll need a compressor map to understand it--and then some.
Pumping ability changes with compressor speed (and in a nonlinear way!). Below about 40,000 RPMs, boost pressure is off-the-map low because centrifugal compressor pumping is an exponential function (at first, almost nothing, eventually tons). The compressor has to accelerate from essentially zero to 40,000 RPMS before you see any boost on the gauge at all.
And everything depends on everything else: Compressor air flow depends on compressor size and trim, speed and discharge pressure. Compressor speed depends on compressor discharge pressure and air flow, and available turbine energy. Air flow depends on instantaneous compressor thermal efficiency, engine displacement, engine volumetric efficiency, engine speed, intercooler efficiency, ambient air temperature, and throttle angle, as well as turbine speed and torque. Turbine speed depends on compressor load, exhaust energy, turbine size and trim, turbine nozzle size and leverage, the reactants-to-products ratio of combustion, exhaust pulse speed, exhaust volume, exhaust gas temperature, and exhaust manifold pressure--all of which depend on engine displacement, engine speed, boost pressure, air-fuel ratio, and all of which are changing constantly as the engine speed and rate of acceleration go up and down. And that's not even considering the effect of the wastegate strategy (how and when you bypass exhaust gases around the turbine to prevent overboost).
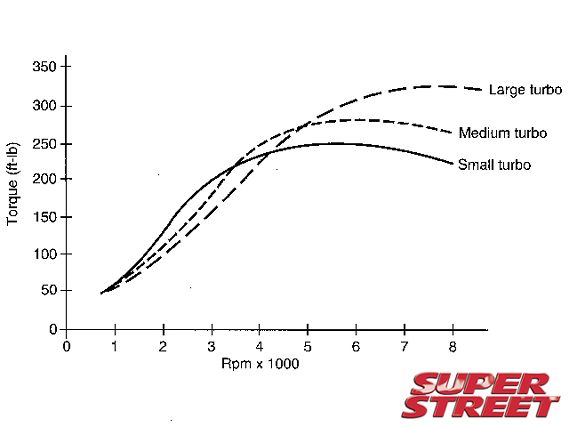
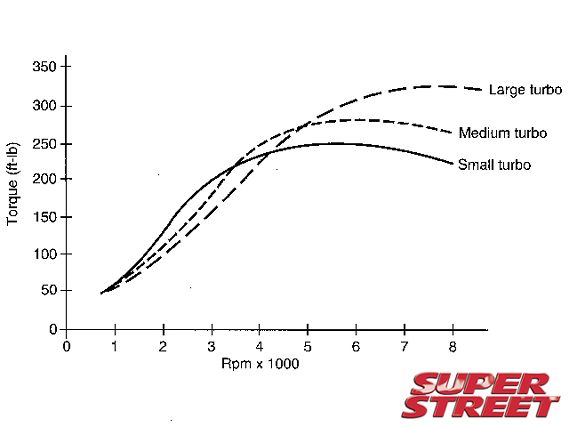
Got that? Take a deep breath and try again: The speed of a compressor depends on how much energy it takes to turn the compressor (a function of manifold pressure, throttle angle, engine volumetric efficiency at RPM, engine RPM and rate of increase in engine RPM, current compressor speed, and rate of increase in compressor speed), available exhaust energy to turn the turbine that's powering the compressor, dynamically increasing exhaust energy from increasing boost pressure (or the reverse), residual heat in the turbine and housing, turbocharger inertia of the complete rotating assembly, friction in the turbocharger (ball bearing or not, oil viscosity, etc), and so forth.
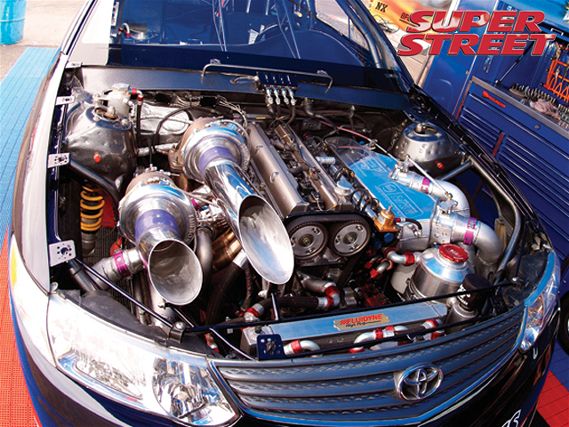
Of course, it's not just pumping (CFM) that determines air mass moving into an engine, it's also intake charge temperature: For a given boost pressure, hotter air is less dense, meaning there's less of it. As you drive, compressor speed and boost pressure move all over the map (literally!), and the thermal efficiency of the turbocharger will vary, as it heats the air more or less. In addition, induction systems, intercoolers, and other components can heat soak. Which tends to diminish air density. The difference between compressor air flow at the first tickle of low-RPM boost and maximum air flow at high compressor speed and high engine VE is tremendous and nonlinear. But as a centrifugal compressor approaches the outer limits of maximum design air flow, it will begin to "choke," meaning the compressor can pump no more air at a given level of boost no matter how fast it turns, but will simply be heating the air with increasing ferocity.
On the low end of air flow, if there is too much boost pressure at too low total mass air flow, the pumping performance can become unstable and the compressor will begin to surge. At this point, the compressor looses its ability to further squeeze the air, which is then free to expand and flow backward as well as forward through the compressor. This "compressor stall" can sound like a sudden "backfire" or series of backfires or "chuffs" of air, with high-pressure air abruptly reversing direction and exploding backward through the compressor wheel in a shock wave that instantly lowers compressor discharge pressure and can damage bearings and damage or destroy the compressor wheel and shaft (and scare the hell out of you from the noise).
Since a turbocharger's freewheeling rotating assembly is able to seek its own speed as compressor and turbine pressures and other factors permit, the turbo is free to out-accelerate the engine (if it can!) and surge ahead to make boost while the engine hunkers down to make more power without necessarily changing RPM a bit. Similarly, just because the engine increases in speed does NOT mean the compressor will accelerate--though it might if engine VE or air flow increase with RPM. Conversely, above peak power, with VE falling, the engine will pump LESS air with RPM increases, which can cause the turbo to slow down and pump less, beginning a negative feedback loop that results in a power-loss death-spiral. Meanwhile, on the high end, if the compressor is small for the engine but the turbine is not yet a bottleneck, if engine VE is increasing with RPM, the turbo might turn so fast it spools up into dangerous above-the-redline overspeed line at the upper right of the compressor map. (Actually you can over-speed a turbo by going too far to the right (in mass flow) OR too far up (in pressure ratio).